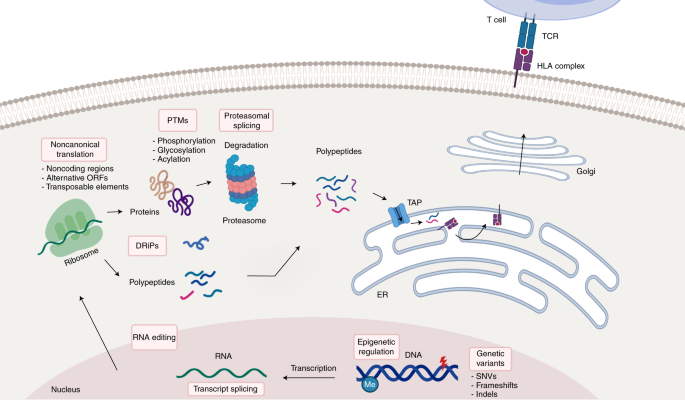
Identification of tumor antigens with immunopeptidomics
Kloetzel, P. M. Antigen processing by the proteasome. Nat. Rev. Mol. Cell Biol. 2, 179–187 (2001).
Coulie, P. G. et al. A mutated intron sequence codes for an antigenic peptide identified by cytolytic T lymphocytes on a human melanoma. Proc. Natl Acad. Sci. USA 92, 7976–7980 (1995).
Roche, P. A. & Furuta, K. The ravishing details of MHC class II-mediated antigen processing and presentation. Nat. Rev. Immunol. 15, 203–216 (2015).
Yewdell, J. W., Reits, E. & Neefjes, J. Making sense of mass destruction: quantitating MHC class I antigen presentation. Nat. Rev. Immunol. 3, 952–961 (2003).
Schumacher, T. N., Scheper, W. & Kvistborg, P. Most cancers neoantigens. Annu. Rev. Immunol. 37, 173–200 (2019).
Bianchi, V., Harari, A. & Coukos, G. Neoantigen-explicit adoptive cell therapies for cancer: making T-cell merchandise more non-public. Entrance. Immunol. 11, 1215 (2020).
Curran, M. A. & Glisson, B. S. Sleek hope for therapeutic cancer vaccines within the period of immune checkpoint modulation. Annu. Rev. Med. 70, 409–424 (2019).
Haen, S. P., Löffler, M. W., Rammensee, H.-G. & Brossart, P. In direction of new horizons: characterization, classification and implications of the tumour antigenic repertoire. Nat. Rev. Clin. Oncol. 17, 595–610 (2020).
Kruger, S. et al. Advances in cancer immunotherapy 2019: most modern trends. J. Exp. Clin. Most cancers Res. 38, 268 (2019).
Christofi, T., Baritaki, S., Falzone, L., Libra, M. & Zaravinos, A. Present views in cancer immunotherapy. Cancers (Basel) 11, 1472 (2019).
Laumont, C. M. et al. Global proteogenomic diagnosis of human MHC class I-associated peptides derived from non-canonical discovering out frames. Nat. Commun. 7, 10238 (2016).
Sebestyen, E. et al. Huge-scale diagnosis of genome and transcriptome alterations in a pair of tumors unveils new cancer-associated splicing networks. Genome Res. 26, 732–744 (2016).
Zhao, Q. et al. Proteogenomics uncovers a wide repertoire of shared tumor-explicit antigens in ovarian cancer. Most cancers Immunol. Res. 8, 544–555 (2020).
Ouspenskaia, T. et al. Thousands of new unannotated proteins prolong the MHC I immunopeptidome in cancer. Preprint at bioRxiv https://doi.org/10.1101/2020.02.12.945840 (2020).
Chen, J. et al. Pervasive purposeful translation of noncanonical human launch discovering out frames. Science 367, 1140–1146 (2020).
Ilyas, S. & Yang, J. C. Panorama of tumor antigens in T cell immunotherapy. J. Immunol. 195, 5117–5122 (2015).
Caballero, O. L. & Chen, Y. T. Most cancers/testis (CT) antigens: doubtless targets for immunotherapy. Most cancers Sci. 100, 2014–2021 (2009).
Tio, D. et al. Expression of cancer/testis antigens in cutaneous melanoma: a systematic evaluate. Melanoma Res. 29, 349–357 (2019).
Schooten, E., Di Maggio, A., van Bergen En Henegouwen, P. M. P. & Kijanka, M. M. MAGE-A antigens as targets for cancer immunotherapy. Most cancers Treat. Rev. 67, 54–62 (2018).
D’Angelo, S. P. et al. Antitumor project associated to extended persistence of adoptively transferred NY-ESO-1 c259T cells in synovial. Sarcoma 8, 944–957 (2018).
Rapoport, A. P. et al. NY-ESO-1–explicit TCR–engineered T cells mediate sustained antigen-explicit antitumor leads to myeloma. Nat. Med. 21, 914–921 (2015).
Robbins, P. F. et al. A pilot trial the utilization of lymphocytes genetically engineered with an NY-ESO-1–reactive T-cell receptor: long-time interval apply-up and correlates with response. Clin. Most cancers Res. 21, 1019–1027 (2015).
Laumont, C. M. & Perreault, C. Exploiting non-canonical translation to establish new targets for T cell-essentially essentially based cancer immunotherapy. Cell. Mol. Lifestyles Sci. 75, 607–621 (2018).
Moreau-Aubry, A. et al. A processed pseudogene codes for a new antigen identified by a CD8+ T cell clone on melanoma. J. Exp. Med. 191, 1617–1623 (2000).
Li, L.-J., Leng, R.-X., Fan, Y.-G., Pan, H.-F. & Ye, D.-Q. Translation of noncoding RNAs: heart of attention on lncRNAs, pri-miRNAs, and circRNAs. Exp. Cell Res. 361, 1–8 (2017).
Charpentier, M. et al. IRES-dependent translation of the long non coding RNA meloe in melanoma cells produces potentially the most immunogenic MELOE antigens. Oncotarget 7, 59704–59713 (2016).
Roulois, D. et al. DNA-demethylating brokers target colorectal cancer cells by inducing viral mimicry by endogenous transcripts. Cell 162, 961–973 (2015).
Chiappinelli, K. B. et al. Inhibiting DNA methylation causes an interferon response in cancer via dsRNA including endogenous retroviruses. Cell 162, 974–986 (2015).
Attermann, A. S., Bjerregaard, A. M., Saini, S. K., Gronbaek, K. & Hadrup, S. R. Human endogenous retroviruses and their implication for immunotherapeutics of cancer. Ann. Oncol. 29, 2183–2191 (2018).
Vigneron, N. et al. An antigenic peptide produced by peptide splicing within the proteasome. Science 304, 587–590 (2004).
Delong, T. et al. Pathogenic CD4 T cells in kind 1 diabetes sign epitopes formed by peptide fusion. Science 351, 711–714 (2016).
Yewdell, J. W. & Holly, J. DRiPs collect molecular. Curr. Opin. Immunol. 64, 130–136 (2020).
Welters, M. J. et al. Induction of tumor-explicit CD4+ and CD8+ T-cell immunity in cervical cancer patients by a human papillomavirus kind 16 E6 and E7 long peptides vaccine. Clin. Most cancers Res. 14, 178–187 (2008).
Morgan, R. A. et al. Most cancers regression and neurological toxicity following anti-MAGE-A3 TCR gene remedy. J. Immunother. 36, 133–151 (2013).
Skipper, J. C. et al. Mass-spectrometric evaluate of HLA-A*0201-associated peptides identifies dominant naturally processed forms of CTL epitopes from MART-1 and gp100. Int J. Most cancers 82, 669–677 (1999).
Wolf, B. et al. Security and tolerability of adoptive cell remedy in cancer. Drug Saf. 42, 315–334 (2019).
Purcell, A. W., Ramarathinam, S. H. & Ternette, N. Mass spectrometry-essentially essentially based identification of MHC-lag peptides for immunopeptidomics. Nat. Protoc. 14, 1687–1707 (2019).
Caron, E. et al. Prognosis of essential histocompatibility complex (MHC) immunopeptidomes the utilization of mass spectrometry. Mol. Cell. Proteom. 14, 3105–3117 (2015).
Ritz, D., Kinzi, J., Neri, D. & Fugmann, T. Records-just acquisition of HLA class I peptidomes on the Q exactive mass spectrometer platform. Proteomics 17, 1700177 (2017).
Gillet, L. C. et al. Targeted recordsdata extraction of the MS/MS spectra generated by recordsdata-just acquisition: a new theory for consistent and appropriate proteome diagnosis. Mol. Cell. Proteom. 11, O111.016717 (2012).
Brunner, A.-D. et al. Extremely-high sensitivity mass spectrometry quantifies single-cell proteome adjustments upon perturbation. Preprint at bioRxiv 2020.2012.2022.423933 (2020).
Tsou, C. C. et al. DIA-Umpire: comprehensive computational framework for recordsdata-just acquisition proteomics. Nat. Methods 12, 258–264 (2015).
Muntel, J. et al. Surpassing 10 000 identified and quantified proteins in a single bustle by optimizing most modern LC-MS instrumentation and recordsdata diagnosis technique. Mol. Omics 15, 348–360 (2019).
Gessulat, S. et al. Prosit: proteome-wide prediction of peptide tandem mass spectra by deep discovering out. Nat. Methods 16, 509–518 (2019).
Croft, N. P. et al. Kinetics of antigen expression and epitope presentation for the interval of virus an infection. PLoS Pathog. 9, e1003129 (2013).
Hassan, C. et al. Exact quantitation of MHC-lag peptides by application of isotopically labeled peptide MHC complexes. J. Proteom. 109, 240–244 (2014).
Croft, N. P., Purcell, A. W. & Tscharke, D. C. Quantifying epitope presentation the utilization of mass spectrometry. Mol. Immunol. 68, 77–80 (2015).
Tan, C. T., Croft, N. P., Dudek, N. L., Williamson, N. A. & Purcell, A. W. Reveal quantitation of MHC-lag peptide epitopes by selected reaction monitoring. Proteomics 11, 2336–2340 (2011).
Kapp, E. A. et al. An evaluate, comparability, and appropriate benchmarking of several publicly available MS/MS search algorithms: sensitivity and specificity diagnosis. Proteomics 5, 3475–3490 (2005).
Kapp, E. & Schutz, F. Overview of tandem mass spectrometry (MS/MS) database search algorithms. Curr. Protoc. Protein Sci. 49, 25.2.1–25.2.19 (2007).
Elias, J. E. & Gygi, S. P. Goal-decoy search technique for increased self assurance in immense-scale protein identifications by mass spectrometry. Nat. Methods 4, 207–214 (2007).
Zhang, J. et al. PEAKS DB: de novo sequencing assisted database survey ravishing and appropriate peptide identification. Mol. Cell. Proteom. 11, M111.010587 (2012).
Shan, P. & Tran, H. Integrating database search and de novo sequencing for immunopeptidomics with DIA method. J. Biomol. Tech. 30, S23 (2019).
Faridi, P., Purcell, A. W. & Croft, N. P. In immunopeptidomics we desire a sniper as an different of a shotgun. Proteomics 18, e1700464 (2018).
Thompson, A. et al. Tandem mass tags: a new quantification technique for comparative diagnosis of complex protein combinations by MS/MS. Anal. Chem. 75, 1895–1904 (2003).
Pfammatter, S. et al. Extending the comprehensiveness of immunopeptidome analyses the utilization of isobaric peptide labeling. Anal. Chem. 92, 9194–9204 (2020).
Ramarathinam, S. H. et al. A peptide-signal amplification technique for the detection and validation of neoepitope presentation on cancer biopsies. Preprint at bioRxiv https://doi.org/10.1101/2020.06.12.145276 (2020).
Stopfer, L. E., Mesfin, J. M., Joughin, B. A., Lauffenburger, D. A. & White, F. M. Multiplexed relative and absolute quantitative immunopeptidomics finds MHC I repertoire alterations precipitated by CDK4/6 inhibition. Nat. Commun. 11, 2760 (2020).
d’Atri, V. et al. Adding a new separation dimension to MS and LC–MS: what’s the utility of ion mobility spectrometry? J. Sep. Sci. 41, 20–67 (2018).
Pfammatter, S. et al. A new differential ion mobility gadget expands the depth of proteome protection and the sensitivity of multiplex proteomic measurements. Mol. Cell. Proteom. 17, 2051–2067 (2018).
Pfammatter, S., Bonneil, E. & Thibault, P. Improvement of quantitative measurements in multiplex proteomics the utilization of high-discipline uneven waveform spectrometry. J. Proteome Res. 15, 4653–4665 (2016).
Meier, F. et al. Online parallel accumulation-serial fragmentation (PASEF) with a new trapped ion mobility mass spectrometer. Mol. Cell. Proteom. 17, 2534–2545 (2018).
Nesvizhskii, A. I. Proteogenomics: ideas, functions and computational recommendations. Nat. Methods 11, 1114–1125 (2014).
Zhang, M. et al. RNA editing derived epitopes plan as cancer antigens to elicit immune responses. Nat. Commun. 9, 3919 (2018).
Wei, Z. et al. The landscape of tumor fusion neoantigens: a pan-cancer. Anal. iScience 21, 249–260 (2019).
Löffler, M. W. et al. Multi-omics discovery of exome-derived neoantigens in hepatocellular carcinoma. Genome Med. 11, 28 (2019).
Kalaora, S. et al. Exercise of HLA peptidomics and entire exome sequencing to establish human immunogenic neo-antigens. Oncotarget 7, 5110–5117 (2016).
Bassani-Sternberg, M., Pletscher-Frankild, S., Jensen, L. J. & Mann, M. Mass spectrometry of human leukocyte antigen class I peptidomes finds solid outcomes of protein abundance and turnover on antigen presentation. Mol. Cell. Proteom. 14, 658–673 (2015).
Khodadoust, M. S. et al. Antigen presentation profiling finds recognition of lymphoma immunoglobulin neoantigens. Nature 543, 723–727 (2017).
Bassani-Sternberg, M. et al. Reveal identification of clinically associated neoepitopes supplied on native human melanoma tissue by mass spectrometry. Nat. Commun. 7, 13404 (2016).
Binz, P. A. et al. Proteomics Standards Initiative extended FASTA structure. J. Proteome Res. 18, 2686–2692 (2019).
Eng, J. K. & Deutsch, E. W. Extending comet for global amino acid variant and put up-translational modification diagnosis the utilization of the PSI extended FASTA structure. Proteomics 72, e1900362 (2020).
Elias, J. E. & Gygi, S. P. Goal-decoy search technique for mass spectrometry-essentially essentially based proteomics. Methods Mol. Biol. 604, 55–71 (2010).
Gupta, N., Bandeira, N., Keich, U. & Pevzner, P. A. Goal-decoy method and fake discovery payment: when issues may match wicked. J. Am. Soc. Mass Spectrom. 22, 1111–1120 (2011).
Tanner, S. et al. Bettering gene annotation the utilization of peptide mass spectrometry. Genome Res. 17, 231–239 (2007).
Chong, C. et al. Integrated proteogenomic deep sequencing and analytics precisely establish non-canonical peptides in tumor immunopeptidomes. Nat. Commun. 11, 1293 (2020).
Laumont, C. M. et al. Noncoding regions are the principle source of targetable tumor-explicit antigens. Sci. Transl. Med. 10, eaau5516 (2018).
Beautiful, A. C. et al. Intron retention is a source of neoepitopes in cancer. Nat. Biotechnol. 36, 1056–1058 (2018).
Attig, J. et al. LTR retroelement growth of the human cancer transcriptome and immunopeptidome revealed by de novo transcript assembly. Genome Res. 29, 1578–1590 (2019).
Kong, Y. et al. Transposable ingredient expression in tumors is said to immune infiltration and increased antigenicity. Nat. Commun. 10, 5228 (2019).
Shraibman, B., Melamed Kadosh, D., Barnea, E. & Admon, A. HLA peptides derived from tumor antigens precipitated by inhibition of DNA methylation for pattern of drug-facilitated immunotherapy. Mol. Cell. Proteom. 15, 3058–3070 (2016).
Calviello, L. et al. Detecting actively translated launch discovering out frames in ribosome profiling recordsdata. Nat. Methods 13, 165–170 (2016).
Erhard, F. et al. Improved Ribo-seq permits identification of cryptic translation events. Nat. Methods 15, 363–366 (2018).
Ingolia, N. T. et al. Ribosome profiling finds pervasive translation outdoors of annotated protein-coding genes. Cell Obtain. 8, 1365–1379 (2014).
Slavoff, S. A. et al. Peptidomic discovery of short launch discovering out frame–encoded peptides in human cells. Nat. Chem. Biol. 9, 59–64 (2013).
Sarkizova, S. et al. A immense peptidome dataset improves HLA class I epitope prediction across many of the human population. Nat. Biotechnol. 38, 199–209 (2020).
Abelin, J. G. et al. Mass spectrometry profiling of HLA-associated peptidomes in mono-allelic cells permits more appropriate epitope prediction. Immunity 46, 315–326 (2017).
Warren, E. H. et al. An antigen produced by splicing of noncontiguous peptides within the reverse uncover. Science 313, 1444–1447 (2006).
Dalet, A. et al. An antigenic peptide produced by reverse splicing and double asparagine deamidation. Proc. Natl Acad. Sci. USA 108, E323–E331 (2011).
Michaux, A. et al. A spliced antigenic peptide comprising a single spliced amino acid is produced within the proteasome by reverse splicing of a longer peptide fragment followed by trimming. J. Immunol. 192, 1962–1971 (2014).
Hanada, K., Yewdell, J. W. & Yang, J. C. Immune recognition of a human renal cancer antigen via put up-translational protein splicing. Nature 427, 252–256 (2004).
Liepe, J. et al. A immense share of HLA class I ligands are proteasome-generated spliced peptides. Science 354, 354–358 (2016).
Faridi, P. et al. A subset of HLA-I peptides are now not genomically templated: proof for cis- and trans-spliced peptide ligands. Sci. Immunol. 3, eaar3947 (2018).
Liepe, J., Sidney, J., Lorenz, F. K. M., Sette, A. & Mishto, M. Mapping the MHC class I–spliced immunopeptidome of cancer cells. Most cancers Immunol. Res. 7, 62–76 (2019).
Paes, W. et al. Contribution of proteasome-catalyzed peptide cis-splicing to viral focusing on by CD8+ T cells in HIV-1 an infection. Proc. Natl Acad. Sci. USA 116, 24748–24759 (2019).
Faridi, P. et al. Spliced peptides and cytokine-pushed adjustments within the immunopeptidome of melanoma. Most cancers Immunol. Res. 8, 1322–1334 (2020).
Mylonas, R. et al. Estimating the contribution of proteasomal spliced peptides to the HLA-I ligandome. Mol. Cell. Proteom. 17, 2347–2357 (2018).
Rolfs, Z., Solntsev, S. K., Shortreed, M. R., Frey, B. L. & Smith, L. M. Global identification of put up-translationally spliced peptides with neo-fusion. J. Proteome Res. 18, 349–358 (2019).
Erhard, F., Dölken, L., Schilling, B. & Schlosser, A. Identification of the cryptic HLA-I immunopeptidome. Most cancers Immunol. Res. 8, 1018–1026 (2020).
Vigneron, N., Ferrari, V., Stroobant, V., Abi Habib, J. & Van den Eynde, B. J. Peptide splicing by the proteasome. J. Biol. Chem. 292, 21170–21179 (2017).
Dalet, A., Vigneron, N., Stroobant, V., Hanada, K. & Van den Eynde, B. J. Splicing of a ways away peptide fragments occurs within the proteasome by transpeptidation and produces the spliced antigenic peptide derived from fibroblast verbalize ingredient-5. J. Immunol. 184, 3016–3024 (2010).
Henry, V. J., Bandrowski, A. E., Pepin, A. S., Gonzalez, B. J. & Desfeux, A. OMICtools: an informative listing for multi-omic recordsdata diagnosis. Database (Oxford) 2014, bau069 (2014).
Afgan, E. et al. The Galaxy platform for accessible, reproducible and collaborative biomedical analyses: 2018 update. Nucleic Acids Res. 46, W537–W544 (2018).
Nesvizhskii, A. I. et al. Dynamic spectrum quality evaluate and iterative computational diagnosis of shotgun proteomic recordsdata: toward more efficient identification of put up-translational adjustments, sequence polymorphisms, and new peptides. Mol. Cell. Proteom. 5, 652–670 (2006).
Andreatta, M. et al. MS-Rescue: a computational pipeline to prolong the standard and yield of immunopeptidomics experiments. Proteomics 19, e1800357 (2019).
Rolfs, Z., Müller, M., Shortreed, M. R., Smith, L. M. & Bassani-Sternberg, M. Statement on ‘A subset of HLA-I peptides are now not genomically templated: proof for cis- and trans-spliced peptide ligands’. Sci. Immunol. 4, eaaw1622 (2019).
McGranahan, N. & Swanton, C. Clonal heterogeneity and tumor evolution: previous, most modern, and the future. Cell 168, 613–628 (2017).
Marcu, A. et al. The HLA Ligand Atlas. A resource of pure HLA ligands supplied on benign tissues. J. Immunother. Most cancers 9, e002071 (2019).
Schatton, T. et al. Identification of cells initiating human melanomas. Nature 451, 345–349 (2008).
Lang, D., Mascarenhas, J. B. & Shea, C. R. Melanocytes, melanocyte stem cells, and melanoma stem cells. Clin. Dermatol. 31, 166–178 (2013).
Kassiotis, G. & Stoye, J. P. Immune responses to endogenous retroelements: taking the contaminated with the very best. Nat. Rev. Immunol. 16, 207–219 (2016).
Rycaj, K. et al. Cytotoxicity of human endogenous retrovirus K–explicit T cells toward autologous ovarian. Most cancers Cells 21, 471–483 (2015).
Saini, S. K. et al. Human endogenous retroviruses invent a reservoir of T cell targets in hematological cancers. Nat. Commun. 11, 5660 (2020).
Mullins, C. S. & Linnebacher, M. Endogenous retrovirus sequences as a new class of tumor-explicit antigens: an example of HERV-H env encoding solid CTL epitopes. Most cancers Immunol. Immun. 61, 1093–1100 (2012).
Tu, X. et al. Human leukemia antigen-A*0201-restricted epitopes of human endogenous retrovirus W family envelope (HERV-W env) induce solid cytotoxic T lymphocyte responses. Virol. Sin. 32, 280–289 (2017).
Belgnaoui, S. M., Gosden, R. G., Semmes, O. J. & Haoudi, A. Human LINE-1 retrotransposon induces DNA injury and apoptosis in cancer cells. Most cancers Cell Int. 6, 13 (2006).
Scott, E. C. et al. A sizzling L1 retrotransposon evades somatic repression and initiates human colorectal cancer. Genome Res. 26, 745–755 (2016).
Ott, P. A. et al. An immunogenic non-public neoantigen vaccine for patients with melanoma. Nature 547, 217–221 (2017).
Sahin, U. et al. Personalized RNA mutanome vaccines mobilize poly-explicit therapeutic immunity in opposition to cancer. Nature 547, 222–226 (2017).
Ebrahimi-Nik, H. et al. Mass spectrometry pushed exploration finds nuances of neoepitope-pushed tumor rejection. JCI Perception 5, e129152 (2019).
Smith, C. C. et al. Replace tumour-explicit antigens. Nat. Rev. Most cancers 19, 465–478 (2019).
Jackson, R. et al. The translation of non-canonical launch discovering out frames controls mucosal immunity. Nature 564, 434–438 (2018).
Muller, M., Gfeller, D., Coukos, G. & Bassani-Sternberg, M. ‘Hotspots’ of antigen presentation revealed by human leukocyte antigen ligandomics for neoantigen prioritization. Entrance. Immunol. 8, 1367 (2017).
Schittenhelm, R. B. et al. A comprehensive diagnosis of constitutive naturally processed and supplied HLA-C*04: 01 (Cw4)-explicit peptides. Tissue Antigen. 83, 174–179 (2014).
Schuster, H. et al. The immunopeptidomic landscape of ovarian carcinomas. Proc. Natl Acad. Sci. USA 114, E9942–E9951 (2017).
Sarkizova, S. et al. A immense peptidome dataset improves HLA class I epitope prediction across many of the human population. Nat. Biotechnol. 38, 199–209 (2020).
Keskin, D. B. et al. Neoantigen vaccine generates intratumoral T cell responses in Fragment Ib glioblastoma trial. Nature 565, 234–239 (2019).
Shraibman, B. et al. Identification of tumor antigens amongst the HLA peptidomes of glioblastoma tumors and plasma. Mol. Cell. Proteom. 17, 2132–2145 (2018).
Ternette, N. et al. Immunopeptidomic profiling of HLA-A2-particular triple detrimental breast cancer identifies doubtless immunotherapy target antigens. Proteomics 18, 1700465 (2018).